- Get link
- X
- Other Apps
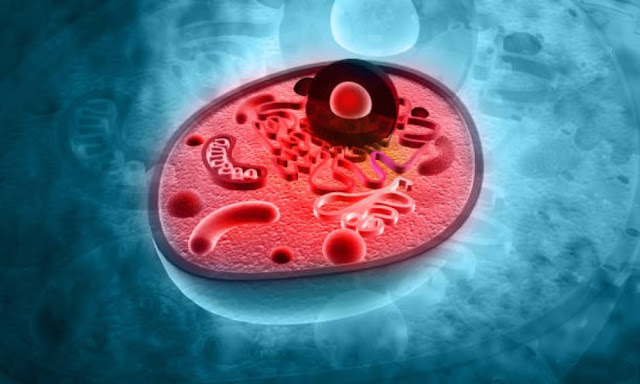
Microscopy technology has revolutionized our understanding
of the microscopic world by enabling the visualization and analysis of
structures and processes at the cellular and molecular levels. Various
microscopy techniques have been developed from traditional light to advanced
electron microscopy, each offering unique capabilities and applications. This
article explores microscopy technology's principles, advancements, and
applications, highlighting its profound impact on scientific research and
technological advancements.
Light Microscopy:
Light microscopy, also known as optical microscopy, is the
most common and accessible form. It utilizes visible light and lenses to
magnify and visualize samples. Key variants of light microscopy include:
Brightfield Microscopy: This technique uses transmitted
white light to visualize stained or naturally pigmented samples. It is commonly
used in biological research and clinical diagnostics.
Fluorescence Microscopy: Fluorescence microscopy employs
fluorescent dyes or proteins to label specific molecules within a sample. It
enables the visualization of cellular structures, protein localization, and
dynamic processes—advanced techniques like confocal and multiphoton microscopy
offer improved resolution and three-dimensional imaging capabilities.
Phase-Contrast Microscopy: Phase-contrast microscopy
enhances the visibility of transparent and unstained samples by converting
phase differences in light waves into contrast. It is widely used in cell
biology and tissue culture.
Electron Microscopy:
Electron microscopy (EM) uses a beam of accelerated
electrons instead of light to obtain higher-resolution images. This technology
allows for the visualization of subcellular structures and nanoscale details.
Key variants of electron microscopy include:
Transmission Electron Microscopy (TEM): TEM employs a beam
of electrons that passes through an ultra-thin sample to create an image. It
provides high-resolution images of internal cell structures, viruses, and
materials at the atomic level.
Scanning Electron Microscopy (SEM): SEM uses a focused beam
of electrons to scan the surface of a sample, generating detailed
three-dimensional images. It is valuable for studying surface morphology,
particle analysis, and materials characterization.
Cryo-Electron Microscopy (Cryo-EM): Cryo-EM is a specialized
technique that enables imaging samples in their native, hydrated state at
cryogenic temperatures. Recent advancements in detector technology and image
processing have led to significant improvements in the resolution and
accessibility of cryo-EM, revolutionizing structural biology.
Scanning Probe Microscopy:
Scanning probe microscopy (SPM) techniques involve scanning
a sharp probe over a sample's surface, measuring various properties to create
high-resolution images. Key variants of SPM include:
Atomic Force Microscopy (AFM): AFM uses a sharp probe to
detect forces between the probe and the sample's surface. It enables the
visualization of surface topography at the atomic and molecular scale and the
mapping of mechanical properties.
Scanning Tunneling Microscopy (STM): STM measures the flow
of electrons between a conductive probe and the sample's surface. It provides
atomic-scale resolution and is commonly used for studying surfaces and thin
films.
Super-Resolution Microscopy:
Super-resolution microscopy techniques surpass the
diffraction limit of light microscopy, allowing for imaging at the nanoscale
level. Special techniques include:
Stimulated Emission Depletion (STED) Microscopy: STED
microscopy uses focused laser beams to selectively deactivate fluorescence
emission, resulting in improved resolution and reduced background noise.
Single-Molecule Localization Microscopy (SMLM): SMLM
techniques, such as PALM and STORM, allow for the precise localization and
reconstruction of single molecules, enabling the visualization of molecular
interactions and cellular structures with nanoscale resolution.
Conclusion:
Microscopy technology has transformed our understanding of
the microscopic world and contributed to numerous scientific discoveries. Each
technique offers unique capabilities and applications, From light to electron
microscopy and scanning probe microscopy. Advances in super-resolution,
correlative, and in vivo imaging have further expanded the boundaries of what
can be visualized and analyzed at the cellular and molecular levels. As
microscopy technology advances, it holds great promise for unlocking new
insights in biology, medicine, materials science, and nanotechnology. The
ongoing developments in hardware, software, and imaging techniques will
continue to drive scientific progress and pave the way for exciting discoveries
in the invisible world.
- Get link
- X
- Other Apps